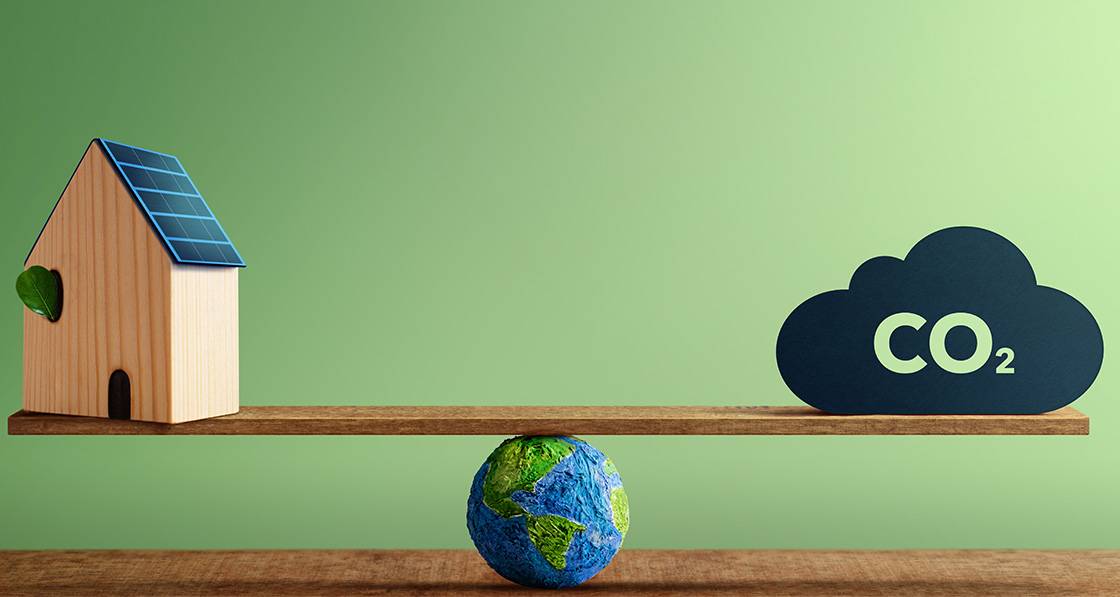

- Insight
- Posted
Much ado about nothing
As the world edges ever closer to the precipice of runaway climate change, some sustainability terms have moved from relative obscurity towards the mainstream of marketing and public discourse – and none more so than zero carbon. But is zero carbon construction a real prospect, or is it just wishful thinking?
Words by John Butler and Andy Simmonds
What is a net zero carbon building? Attempts at a definition are underway in the UK and Ireland
In the UK, a proposal for a net zero carbon building standard is being developed by a group of prominent industry bodies. For more information visit www.nzcbuildings.co.uk/.
A full list of characteristics and metrics for this proposed standard are shown in the technical update and consultation document, including limits on embodied carbon and operational energy, and minimum targets for aspects such as on-site renewables, demand flexibility, for example. You can read the document at: tinyurl.com/ysft3aec.
In Ireland, the IGBC recently held a consultation on a proposed net zero carbon building definition, and is processing the results. This can be read at: tinyurl.com/IGBCnetzerocarbon.
Increasingly claims are made that some buildings are zero carbon in their construction (as opposed to being operationally zero carbon). The incorporation of sequestered biogenic carbon in large quantities of plant-based materials (carbon that has been removed from the atmosphere and stored in the structure of plants while they grow) is seized upon as evidence that the resulting building is zero carbon or even ‘carbon negative’.
Such claims are usually based on the idea that more carbon is stored in the materials used to construct the building than was emitted to create those materials and assemble them into the building.
While this feels like it might make sense the reality is much more complex, making such claims deeply problematic.
Is guidance aligned with standards?
In some areas planning requirements or guidance are being developed to encourage zero carbon development, in both operational and embodied terms. It’s a laudable aim. However, poorly worded guidance can lead to increased misunderstanding of what is really entailed in attempting to achieve genuinely low carbon construction.
The main standards setting out how to calculate the carbon emissions of products and whole buildings state with increasing clarity that the ‘negative emissions’, i.e., the sequestered carbon of biogenic materials, may only be reported for projects when the whole carbon life cycle is accounted for. These key documents are:
- EN15804+A2 ‘Sustainability of construction works: Environmental product declarations - Core rules for the product category of construction products’.
- RICS ‘Whole life carbon assessment (WLCA) for the built environment’.
In fact, EN 15804+A2 puts it the other way around: if a product contains biogenic carbon, then you must account for the whole life cycle. Additionally, it states that the stored carbon cannot be reported for products coming from native forests (carbon emissions resulting from harvesting such resources are reported under “land use and land use change emissions”), and the RICS methodology requires that in the case of timber, only stored carbon in FSC or equivalent certified sustainable forestry can be counted.
A strong carbon buffering argument can be made for systems such as the Ecococon modular straw bale system, which use straw, an annual rotation crop.
Creative accounting
Sticking to the approach set out in the standards is crucial to avoid misrepresenting projects and downright creative accounting.
What do we mean by ‘creative accounting’? For example, suppose the negative figures for stored carbon are added together with the upfront carbon emissions , and it happens to be the case that more carbon is stored in the building than was emitted in delivering the building – as can happen in buildings where there’s very high use of biogenic materials. The stored carbon may appear to cancel out the emissions, and may even be used to claim the building is ‘carbon negative’ – perhaps evoking an emotional concept of it actively sucking carbon out of the sky? While this may look appealing to marketing departments, it simply doesn’t reflect reality. The reality is that the manufacture of any product (including those made from plants) results in some emission of carbon now.
Sticking to a standard approach is also the only way any comparison can be made between projects to aid more focused and effective research and development in the industry.
There is confusion around the importance and timing of emissions. Carbon stored in timber materials will have been absorbed over many decades of growth, and it will take many decades of growth to repeat the process after the timber has been felled, extracted, and used in a building. By contrast, in the case of fast-growing crops used in construction such as straw, sequestration takes place over the previous annual growing season and is regrown just as fast.
As electricity grids and manufacturing industries decarbonise (decarbonising the UK electricity grid is progressing faster than the manufacture of common building materials) then the level of emissions from the manufacturing and processing of material for construction may reduce.
But again, that decarbonisation process is happening over decades, and much is still uncertain – bringing us back to the point that right now pretty much all construction causes immediate emissions to a heavily carbon-polluted atmosphere.
End of life - future planning
It’s also important to note that any biogenic carbon in a product or building will eventually be released at the end of the lifecycle of that product or building. Again, on timing of the carbon ‘leakage’: carbon from badly detailed timber cladding or landscaping features will find its way to the atmosphere quicker than the timber structural elements or natural-fibre based insulations or linings of a well-detailed and well-built building.
Even if a biogenic material is removed from a building and re-used intact in another building, from the carbon-accounting perspective the carbon is still ‘released’ from the first building (which is no longer storing its precious carbon-cargo), and the ‘negative emissions’ value is then recognised in the carbon accounts of the new building. When planning a building we can (and should) design with a clear intent for reuse or recycling of the materials in it, helping future generations (or our future selves) when the building is ultimately deconstructed.
They won’t thank us for buildings and materials that are hard to repurpose, reuse or recycle!
We must accept that we can’t know what will happen however well we design – but that is no excuse not to think long term. Access to resources appears almost certain to be much more difficult in the years ahead. Clearly, to limit the worst impacts of anthropogenic carbon emissions on climate change and make wise use of resources over time, we need to make the fastest reductions in emissions now, and now means within the next ten years. But we still cannot ignore future emissions. It is essential that the potential release of carbon at the end of life is considered.
Genuine low carbon construction means now and in the future
The LETI approach to embodied carbon targets and benchmarking is useful here, where a building must meet targets both for upfront and life cycle embodied carbon. They suggest that by 2030 at the latest we should be building to their A rating, with A+ and A++ still to aspire to – arguably where we should be aiming already.
Band A, applied to residential buildings, allows up to 350 kgCO2e per square metre of gross internal area (GIA) for upfront carbon and 530 kgCO2e/m2 (GIA) for life cycle embodied carbon.
The lower allowance for upfront emissions addresses the need to reduce real-terms emissions right now. The increased allowance for life cycle embodied carbon reflects not just the potential future emissions of the stored carbon from disposal or processing of all materials at the building’s end of life (EOL), but also emissions from product replacements and maintenance during a building’s life.
Biogenic materials
There are many discussions to be had around the storing of carbon long term in building materials, but the main issue is that while many tonnes of carbon might be temporarily locked up in the plant-based materials in a building, they won’t stay locked up forever. Clearly this practical reality is in direct conflict with the simplistic notion of zero carbon construction.
With plant-based materials (and many non-plant-based ones) there are further emissions associated with land use and land use change. Another welcome addition to EN15804 +A2 is that these emissions must now be accounted for and stated separately.
Carbon stored in soil can be released by cultivation. Any existing vegetation removed to make way for crops will result in carbon emissions, and there are also potential biodiversity impacts from land use and land use change.
Figure 1: LETI’s upfront and cradle-to-grave carbon targets
Carbon release buffer
However, there is a case to make that the biogenic carbon in building materials can provide a useful carbon release buffer. Using materials made from plants that reduce the amount of carbon in the atmosphere – and then storing that carbon in buildings even temporarily – may be beneficial as it slows the rate of release of that carbon.
The exact length of storage is uncertain, tied to the future life of that building and any decisions or disasters that may befall it. But when left to decompose, the plant material releases its carbon back to the atmosphere within a few years. Incorporated into a building, it could be locked up for anything from a couple of decades to hundreds of years.
Quality of construction also plays a significant role here, to ensure a building that can last for the longest time possible, and with the fewest product replacements during its life. Ultimately, it will still be released but there is large potential to slow and delay that release in real terms.
Using resources efficiently
As has been clearly written about before this does not mean we should use as much plant-based material as possible. Sensible and efficient use of any resource is critical, to avoid the immediate impacts caused by its production. The potential biodiversity impacts of any material must also be considered; the less material is used, the lower those impacts are likely to be.
Using recycled materials – and designing buildings to enable re-use of materials – also plays an essential role in reducing carbon emissions and extraction of new material.
Whatever type of material is used it is vital to use only the smallest amount necessary to safely, effectively and durably achieve the aim, whether that is structure, insulation, or weather proofing.
Speed of sequestration
Whatever type of material is used it is vital to use the smallest amount necessary to safely, effectively and durably achieve the aim
The speed of growth of a plant used to make a building material needs to be considered, and whether that plant would be more effective at storing carbon if it remained a growing plant.
The common example is timber, or as it’s known before it becomes a building product: trees. A tree is most effective at storing carbon while it remains alive and growing. Only around 50 per cent of the carbon stored by a growing tree is contained within the part of the stem used to produce timber products. The rest is released back into the system through decomposition or combustion after harvest, though there does also appear to be a degree of increased carbon storage in rotation-cropped forest land over time. The carbon that is stored in trees has been absorbed slowly over decades – but can all be released in one burst at the end of life of a building.
This points strongly to the use of annual – or perennial but annually harvested – crops wherever possible (with longer rotation crops such as trees only used where necessary, such as to provide structure, and in the smallest quantity necessary to safely and effectively do the required job). Such annual crops have stored all their carbon in the growing season immediately preceding their harvest. The carbon stored in the residue from these crops is frequently released back to the atmosphere within a short cycle – for example where the residue is allowed to rot or is burned (or used as animal bedding and then allowed to decompose, etc.).
Locking such plant materials in a building prevents that release and keeps the carbon stored for the life of a building.
Where a building efficiently uses plantbased materials from annual-growth crops in place of non-plant-based materials, it has maximised the storage of recently and rapidly absorbed carbon. Such a building is making the most of its potential to contribute to that useful buffering of atmospheric carbon. Ultimately though, it isn’t, and can’t be. That stored carbon will always be released from the building in the end.
There is no zero impact building We need the construction sector to get better at understanding this. Any building results in emissions and biodiversity impacts. We should always seek to minimise those first and foremost.
We need to think in terms of carbon stewardship in building design and material choices. The choices we make need to reduce atmospheric carbon now, and consider what will happen to stored carbon throughout the life cycle of a building and beyond. Only once that is done can the amount of sequestered carbon be stated as a separate figure (an indicator of the amount of atmospheric carbon buffering that building provides), with the understanding that however large a negative number it may technically be – and whatever the lifespan of that building – the carbon is only held temporarily within its structure.
What about offsetting?
This understandably remains a controversial area. Even if the principle of offsetting (absorbing carbon in one place to offset or balance carbon emitted elsewhere) is accepted, it must never be used to excuse excessive resource use. But, if resources have been used as efficiently as possible and plant-based materials are used, is there a case for then offsetting the residual emissions that do occur?
As laid out above, storing carbon from rapid-rotation plant materials in a building can provide a useful delay to that carbon’s release. It cannot be used to offset the emissions caused by its construction, as that stored carbon will ultimately be released.
Unrealistic scale
A residential building of 120 m2 GIA achieving a LETI ‘A’ rating for both upfront and life cycle embodied carbon would result in emissions of around 42 tonnes of upfront carbon, with a further 21.6 tonnes emitted by the end of life of that building.
Across Europe and the UK, the average annual carbon sequestration rate across different types of forest is 3.2 tonnes CO2 per hectare per year.
So, it would take a hectare of average European forest 13 years to absorb the upfront emissions of the relatively low carbon house described above. In case that doesn’t sound too bad, consider that 204,530 houses were built in the UK in 2022. Even if all of these were somehow achieving LETI ‘A’ rating for upfront carbon emissions, that would amount to 8.6 million tonnes of CO2 released in one year. To sequester those new emissions within the same 13 years would require one hectare of new forestry per house – or rather an equivalent amount reaching sufficient maturity every year to provide the required level of sequestration. That’s an area 1.3 times the size of Greater London requiring forestation every year, just to ‘keep up’ with the annual upfront emissions of housing. And that’s ignoring emissions resulting from external landscaping, access, infrastructure etc., which could be significant in their own right – not to mention non-domestic construction.
This is absolutely not to suggest that we shouldn’t plant trees or increase other crucial means of drawing carbon from the atmosphere such as peat bog restoration or rewilding (which also increase biodiversity), but it highlights the scale of offsetting necessary.
Uncertainty
Calculating the type and size of forestation needed in this theoretical scenario is complicated. The figures above are based on average sequestration rates across Europe, and forestry of average age. Outside the averages, generally the rate of tree biomass growth – and of carbon sequestration – increases with the size of tree but there is some evidence that the rate of sequestration is reduced in forests of mature big trees , partly due to there being fewer trees per area of mature forest. In theory there may come a point in the life cycle of a tree in a managed forest whereby it will continue to store a greater amount of carbon if removed from the forest, making way for new younger trees at a greater density. But cutting that mature tree down also causes carbon emissions, from the unused biomass (roots, offcuts) and from disturbed soil. There is other evidence that reducing forest, management increases the amount of sequestration in that forest however the same study found that ceasing all management of forests would only offset four years of global carbon emissions.
The situation is further complicated by a changing climate affecting the stability and predictability of carbon stored in land and forestry. As figure 2 shows, removals of carbon from all land-based sources in the EU (figure below the line) have been falling overall in recent years with a marked decrease in carbon sequestered in forest land. Currently this trend is predicted to continue, though perhaps with different management and planting policies it could be improved.
The Czech Republic has been hit by what the UNFCCC describes as “extreme drought-induced accelerating bark-beetle outbreak calamity (since 2015)”, resulting in land-use, land-use change, and forestry (LULUCF) emissions going rapidly from 6,964 tonnes of CO2e stored in 2015 to 11,268 tonnes emitted in 2020.
Other countries have seen an increase in bark beetle activity too, and with changing climatic conditions it could spread further.
Figure 2: EU emissions and removals of the LULUCF sector by main land use category. Source: EEA
It's complicated
The point is: it’s complicated and problematic to assess how effective offsetting is, or how much offsetting is required. Basic calculations indicate huge areas of forestry would be necessary just to provide carbon sequestration facilities. Although management of these areas could provide some timber for construction, presumably further additional areas would be required to provide for increasing use of timber in construction.
Again: any materials we use must be used as efficiently as possible. Offsetting at the speed needed to avoid emissions now and within the next 10 years is unrealistic. Which leads to difficult questions about what we should be building, and how.
Our recommendations
- Carbon emissions at any life cycle stage must be reported in line with existing standards: EN15978, EN15804+A2, RICS Whole Life Carbon Assessment in the Built Environment.
- Report kg CO2/m2 and total tonnes emissions. Additionally reporting tonnes emissions per occupant would encourage efficient sufficiency and sensible use of space.
- Stored carbon must never be included in a net figure when reporting upfront carbon (life cycle stages A1-A5, or ‘cradle to practical completion’ of a building). It must only be reported as per the standards/guidance above, i.e.: as a separate figure only.
- There should be targets for upfront and life cycle carbon in any local or national guidance, with both targets requiring to be met.
- These targets should be rapidly reduced to the smallest level to ensure the most rapid decarbonisation possible.
- LETI A+ and A++ should not be considered future aspirational targets but as a representation of where we urgently need to be.
- Retrofit before newbuild. Where demolition is proposed, whole life carbon calculations (combined embodied and operational emissions) must be able to clearly demonstrate that a new building will have a lower carbon impact than a retrofit of the existing building to provide the same floor area as the proposed newbuild.
- Report rapidly sequestered biogenic carbon separately from that in timber products.
- Rapid growth biogenic materials should substitute higher carbon materials effectively, not be used in excessive amounts just to claim a greater stored carbon credit.
- Explore ways of rewarding carbon release buffering resulting from use of rapid- growth/rotation biogenic materials and their associated carbon storage – whilst ensuring that materials are only used as efficiently as possible.
- Operational energy and resulting emissions must be reduced radically alongside reducing embodied emissions.
- External offsetting should never be used as a substitute for reducing actual emissions from construction to the lowest level possible. Whilst there are planting, land use, and forestry practices that can increase stored carbon, there are many uncertainties involved. Such schemes need to be carefully planned to ensure they are genuinely effective (e.g., tree planting in the right place, on poor quality land with low prior carbon retention; compared to tree planting in the wrong place where it can lead to increased emissions from land that previously had high carbon storage value.
- Maximise potential to use recycled materials in new construction or retrofit and to enable reuse and recycling of building components at the end of life of a building.