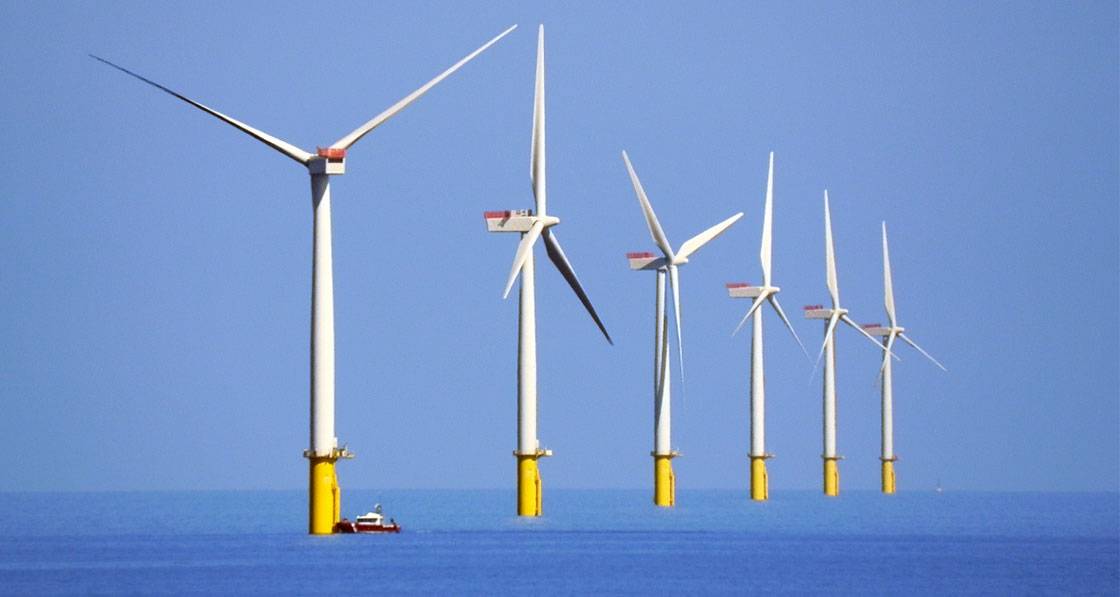
- Blogs
- Posted
How will we decarbonise heating?
Insulating our homes is critical and must be our first priority, but how do we get the rest of the way to zero carbon? Dr Peter Rickaby investigates the options…
This article was originally published in issue 39 of Passive House Plus magazine. Want immediate access to all back issues and exclusive extra content? Click here to subscribe for as little as €10, or click here to receive the next issue free of charge
We are committed to ‘net zero carbon’ by 2050. The use of fossil fuels for space and water heating, and for cooking, will not be permissible. There has been much promotion of decarbonisation options, but it is important to distinguish opportunities from myths.
It is also important that we are not distracted by questions to which reliable answers will not be known for some time. Instead, we should be making progress in a ‘no regrets’ direction, while keeping options open. Questions to which the answers are not yet known include:
- What will the emissions factor for electricity be in 2050?
- How would local electricity networks cope with the electrification of heat?
- What will the penetration of heat networks be?
- To what extent will hydrogen be available via the gas network?
- What will be the characteristics and costs of battery packs?
- How might ‘smart homes’ technology help match supply and demand?
We do know that demand reduction is an essential feature of all options. The no regrets strategy is therefore:
- Improve the building fabric to reduce heat demand.
- Improve the building services to satisfy the remaining demand efficiently, and remove fossil fuel appliances.
- Top-up performance to zero carbon when more is known about the residual emissions from electricity, and about other options.
This approach gives us a little time to deliver stage one, while working out the answers to the questions above so we can decarbonise heat at stage two. If grid electricity is not completely decarbonised, stage three may involve local renewable energy systems and storage.
However, Lowe and Oreszczyn (2020) point out that a convex trajectory to zero carbon may involve twice the emissions between now and 2050 than are associated with a concave trajectory in which early progress is made, so there is a case for prioritising decarbonisation as well as reducing demand (see diagram below).
Sketch of concave and convex carbon dioxide emissions reduction trajectories (source: Lowe and Oreszczyn 2020).
In the average UK dwelling, 87 per cent of energy use is for space heating, hot water and cooking. Homes use nearly four times as much energy in the form of heat as in the form of power. The most frequently discussed option for decarbonising heat is to ‘electrify’ it, i.e., change to appliances such as heat pumps. This does not at present deliver decarbonisation: the emissions factor for electricity is similar to that for gas. However, progress is being made towards the decarbonisation of electricity by replacing fossil-fuelled generation with renewable systems such as wind power. Currently, the cheapest source of electricity supplied to the grid is offshore wind.
If we convert all heat demand to electricity demand, we will need the equivalent of nearly thirty times as much wind power supplying the grid as we have now. If we use heat pumps with an average CoP of 2.5 we will still need the equivalent of twelve times as much wind power. If we adopt the ‘fabric first’ approach described above and reduce demand by 60 per cent, we should be able to reduce the factor from twelve to four. This emphasises how important demand-reduction via retrofit is to decarbonisation.
Another problem is that local electricity distribution networks do not have sufficient capacity to cope with a four-fold increase in demand. This is exacerbated by the transition to electric vehicles, which may increase demand by a further factor of three. This emphasises management and the potentially transformative effect of local energy storage smoothing out the supply- demand mismatch.
Using hydrogen for heating makes little sense.
Electrifying heat is not a decarbonisation panacea, but it has a contribution to make, enabled by retrofit. Where we do it, there are technical considerations. Heat pumps supply heat at a relatively low temperature: typically, between 40 C and 50 C, therefore, for heat distribution within a home a larger radiator area is needed, or underfloor heating. An air source heat pump will struggle to cope with the demand of a poorly insulated house, especially during a prolonged cold snap; it is therefore essential to reduce demand to a level that the heat pump can satisfy, by improving insulation.
Electricity currently costs more than gas, so unless homes are well insulated householders will see their costs rise, and fuel poor households will be disadvantaged. Tariff reform would help to address this: currently in the UK public subsidies are applied to gas, but energy efficiency levies are applied to electricity. This is the reverse of the arrangement we need to promote decarbonisation.
Another frequently discussed option is connecting homes to local heat networks. Developing heat networks in urban areas (they are not viable in rural areas) is expensive and disruptive, and the supply has to be matched to demand. Small scale networks for blocks, estates or mixed-use developments have a part to play. If we can establish heat networks, the challenge is to secure zero carbon heat for them to supply. Sources include waste, biomass and hydrogen, but supplies are limited.
The potential extent of heat networks is not clear, but networks have a contribution to make by reducing demand on the electricity grid. Where networks are developed, the connection of new developments and retrofit schemes may become mandatory.
Much has been claimed about replacing the natural gas that we distribute to our homes with hydrogen. When hydrogen is burned the only combustion product is water, so this is an attractive option. The problem is how to make the hydrogen; there are three methods:
- Grey’ hydrogen is manufactured from fossil fuels, with substantial emissions.
- ‘Blue’ hydrogen is manufactured the same way but with carbon capture and storage, a technology that has so far proven elusive and expensive.
- ‘Green’ hydrogen is made by electrolysing water, so green hydrogen can be zero carbon if the electricity is zero carbon. Essentially this means wind power.
The question raised by the green hydrogen option is why would we use electricity from wind to make hydrogen, then send the hydrogen to homes to be burned in 90 per cent efficient boilers, when we could send the electricity to the homes instead and use it in heat pumps at 250 per cent efficiency? Delivering 1.0 joules (J) of heating requires approximately 1.2 J of natural gas, or 1.6 J of electricity to make 1.2 J of green hydrogen; but only 0.4 J of electricity is needed to deliver 1.0 J of heat via a heat pump. Thus, using hydrogen for heating makes little sense, and the emerging role of hydrogen is as an industrial fuel, for large vehicles and for energy storage. Hydrogen heating seems unlikely to become common.
Insulating homes, heat pumps, and solar PV can all be part of the mix of solutions towards decarbonising our heating systems.
Local solar systems have a role to play. Electricity from solar PV can be stored in batteries or used for water heating, or solar thermal systems can be used to make hot water. In both cases the solar systems can raise the tank temperature and reduce the load on the heat pump. In the UK, a solar thermal system can typically supply half of a dwelling’s annual hot water demand with no emissions. However, the solar input is insufficient in winter, so another system must also be in place.
For cooking, replacing gas-fired hobs with electric hobs is straightforward. However, electric hobs are not as controllable as gas hobs, and electricity is more expensive than gas, so this change may be resisted. Electric induction hobs are more controllable, but they are expensive; prices may fall as demand increases.
What can we conclude? Decarbonising heat is not easy, but it is urgent. There are several options, none of which is yet viable as a generic solution, but some of which will have a role to play. For urban areas with zero carbon heat networks, connection is probably the best option. Elsewhere, using electric heat pumps seems a good option, provided we can decarbonise the supply and increase local distribution capacity. Hydrogen heating makes little sense and seems unlikely to become widespread. While these options are being developed, the ‘no regrets’ approach is to focus on insulating our buildings, in order to reduce the demand for heat as much as possible
Reference Lowe, R. and Oreszczyn, T. (2020) Building decarbonisation transition pathways: initial reflections. CREDS Policy brief 013. Oxford, UK: Centre for Research into Energy Demand Solutions.