- Renewable Energy
- Posted
Search for a store
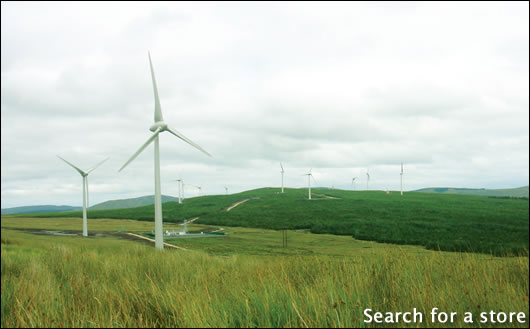
The intermittency of wind is a well-known problem facing Ireland’s most abundant green energy source. Richard Douthwaite explores the best options available to solve the intermittency problem and help Ireland to harness its wind resources.
It is confidently expected that Ireland will get 40% of its electricity from the wind by 2020. This will create an enormous problem as that 40% is an average figure over the year, and there will be lots of days on which there's little or no wind. Consequently, between 120 and 150% more electricity than the country normally consumes will be produced at times when the wind is blowing strongly. Unless ways of disposing of that wind power are found, all other types of power generation will have to be turned off to make room for it and even some of the wind turbines themselves will have to be stood down.
Indeed, the problem has surfaced already. Around half of the country's electricity was produced from the wind at a time of low demand in April and similar levels have been achieved briefly this autumn. During these periods, some gas-fired power stations have had to reduce output and, as they were designed to run at full capacity, that meant that they operated inefficiently, consuming more gas per kWh produced.
Although on occasion some of the wind stations have been told not to produce, there was even a time recently when the fossil generators were so reluctant to reduce the output from their plants only to have to increase them to meet an expected peak in demand a few hours later, that they actually paid the grid to take their power. As a result, big power consumers whose bills are based on the wholesale price for electricity set every 30 minutes by the all-Ireland Single Electricity Market's rolling auction system, were paid to take power too.
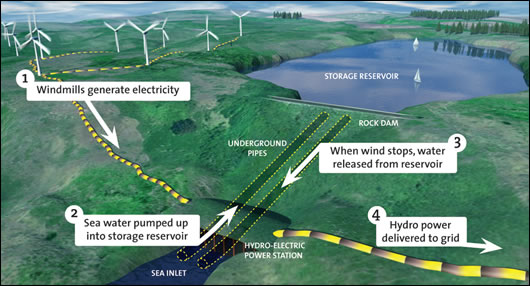
A diagram showing how pumped storage technology operates using the Spirit of Ireland’s idea of utilising the sea as the lower resevoir
These difficulties can only get worse as more windfarms open but, fortunately, several solutions exist. They will be used in combination with each other but it's not yet clear what that combination will be. The aim of this article is to explore the possible outcomes. Let's look at each potential solution in turn.
Possible solution 1: export the surplus power
This is the solution favoured by two of the founders of Airtricity – Eddie O'Connor and Brian Hurley – who believe that as wind power develops throughout Europe, every country will find that there are times when it has too much and times when it has too little. However, these gluts and shortages will not occur at the same time because the whole of the continent will not be in the same weather system. Airtricity carried out a study which showed that if a network of offshore windfarms was built off every coast from the West of Ireland to the Baltic, there would be very few occasions when wind power was not being produced somewhere. The trick is therefore to build enough interconnectors to take the surpluses to the countries where the wind is not blowing, which is exactly what the proposed European Super Grid would do.
That grid will come too late to do anything for Ireland in the next decade. Even Eirgrid's 500MW interconnector which will be completed in 2012 will only help to a limited extent. Work started on it in July and it will run from Rush North Beach, Co Dublin, to Barkby Beach in North Wales. The reason why it will only be of limited help is that wind output can fall off very rapidly. A modelling exercise on recordings from the Met Eireann observatory in Belmullet shows that for 1800MW of wind farms, output could fall from 1800MW to 200MW within an hour.
Dr Pat O'Donoghue who advises the Spirit of Ireland project points out that it is much more difficult to compensate for a drop in wind than for a sudden increase because, with an increase, turbines can be switched off whereas a drop in wind means either switching off customers or switching on unused fossil generating plant.
“This 1600MW drop would require a compensating 1600MW of alternative plant to be brought on line to maintain steady output,” he says. “This would be incredibly difficult. It is more than the entire installed capacity of the fast-reacting open cycle gas turbine (OCGT) plant in the country at present.”
O'Donoghue thinks that, because of delays in replacing Britain's aging nuclear stations and the likely closure of some of its coal stations when phase three of the EU Emissions Trading Scheme pushes up the cost of using coal after 2013, Britain will be so short of power that the interconnector will be used mostly to supply it with electricity.
“If wind suddenly falls off in Ireland, Eirgrid will have to stop exports to the UK to avoid disaster at home,” he says. “Besides losing the export revenue it will have to pay fines for not meeting its half-hour supply contracts. It could try to import power from Britain to meet its shortfall, but the UK National Grid is extremely unlikely to be able to send 500MW to Ireland quickly after being left short of the 500MW Ireland was due to export.”
O'Donoghue admits that such a massive rapid swing in wind output would be a rare event but argues that the grid operators need to have the capacity to meet rare and unexpected challenges. And that, for him, means electricity must be stored although he hastens to point out that interconnection has an important role to play, especially as Spirit of Ireland expects to export power.
Possible solution 2: storing the surplus power
Four possible power storage technologies are vying for adoption. These are pumped storage, flow batteries, compressed air and an attractive newcomer, gravel batteries. Let's look at the pros and cons of each.
Pumped storage, the solution adopted by Spirit of Ireland, is old technology, already in use in Ireland at Turlough Hill. All it involves is pumping water up to a high-level reservoir when power is cheap and running the water back down through a turbine when the power is expensive. Roughly 30% of the energy is lost in the process but its big advantage is that it can switch from storing to supplying in seconds, whereas OCGT plant takes around 15 minutes to start up and coal plants like Moneypoint need many hours to come to full load from cold.
This gives it a big advantage in coping with sudden fluctuations according to O'Donoghue. “If a 100MW pumped storage plant is pumping at full capacity, it can first drop its 100MW pumping load, which can ease the potential wind drop off problem,” he says. “It can then be switched to generating mode and produce 100MW output to further compensate for the loss of wind output. Hence, a 100MW pump/turbine produces a 200MW advantage to compensate for a fall off in wind.”
Normally, pumped storage is costly because a typical installation involves building two reservoirs, one at low level, the other much higher up and linking them with the pipe feeding the pump/turbine. Spirit of Ireland's bright idea is to reduce these costs to a minimum by using the sea as the lower reservoir and finding suitable valleys on the West of Ireland coast so that it is only necessary to install the equipment and to build dam walls across their mouths. A number of possible sites are undergoing detailed evaluation and the Spirit of Ireland team expect to be able to build a pumped storage reservoir and 1000MW power station for €850 million. This makes the cost per megawatt – €.85 million – comparable with those anticipated by most of the over 7GW of new pumped storage plants being planned elsewhere in Europe. However, the Sustainable Energy Research Group at UCC found that European costs were low because in most cases they were planning to adapt existing resources or had one reservoir already in place.
Flow batteries consist of two pairs of two tanks containing liquid chemicals and a reaction cell which is divided in two by a semi-permeable membrane. During the charging cycle, the two electrolytes are pumped slowly through their respective sides of the reaction cell and a current is passed from an electrode on one side of the membrane to an electrode on the other. This changes both the electrolytes chemically. They are then stored in the tanks on the far side of the reaction cell until power needs to be recovered. When it is, the electrolytes are pumped back through the reaction cell and the chemical changes are reversed, producing an electric current, and the spent electrolytes are returned to their original tanks to be re-used on the next charging cycle.
A few years ago it looked as though flow batteries would be adopted widely because it was possible to build them any size you liked. Indeed, the only thing which determined how much energy you could store was the size of the electrolyte tanks and you could increase output by adding additional reaction cells. Now, however, since VRB Power Systems of Vancouver collapsed and had its intellectual assets bought up by a Chinese company, Prudent Energy, in 2009, the technology seems to be dying on its feet. VRB was the main company promoting vanadium redox batteries, the type considered most promising for power-company scale storage. It was to have supplied a 2MW unit for the Sorne Hill wind farm in Donegal at the very high price of €3 million/MW. Sorne Hill might have been the largest of the very few flow battery installations in the world. The existing ones range in size from 10kW to 1.5MW.
The same amount of the electricity, 30%, is lost during the storage and recovery process in a flow battery as with pumped storage. However, although an installation has a life expectancy of at least 30 years, it is not likely to last as long as a dam and turbine. Consequently, flow batteries will not go into widespread use unless their cost can be brought right down.
Compressed air energy storage (CAES). A Dublin-based firm, Gaelectric, plans to carry out drilling near Larne next year to assess whether the salt beds there are suitable for storing compressed air. If the results are promising, it plans to create caverns in the beds by dissolving some of the salt with water. “The technique has been used across Europe and the United States for over 50 years,” says Keith McGrane, Gaelectric's head of energy storage. “The plant we are planning could act as a system balancing facility, putting excess wind energy into storage and re-delivering that to the grid when it is needed.”
The fact that Gaelectric needs to make a store for the air highlights one of the constraints with CAES – it cannot be done everywhere and there is a cost involved in developing a store. It has been suggested that disused gas fields such as that off Kinsale could be used for CAES but not only has this yet to be demonstrated – in many cases, the size of the reservoir might be too large for the air to be kept at the desired pressure. “Gaelectric is hoping to demonstrate CAES in a depleted gas field in Montana. This would be a world first,” McGrane says. “The challenge is to ensure that the air does not react with the gases in the reservoir. An explosive mixture could make the structure unusable for air cycling and storage.”
Using a depleted gas field would transform CAES. When the air is compressed, it heats up. Of the only two CAES plants in operation so far – a 300MW plant at Hundorf in Germany built in 1978 and a 110MW plant in McIntosh, Alabama, built in 1991 – both waste the large amounts of heat generated. When they allow the stored compressed air to expand, it cools down. To counteract this, they mix it with natural gas and burn the mixture in the turbine. This takes a third of the gas which a conventional gas turbine would use for the same output. The thermodynamic efficiency of this process is around 54%. Any residual gas from a depleted well would obviously raise this figure.
Adiabatic CAES stores the heat given off during compression to use during expansion. It avoids the need to use natural gas and is expected to achieve efficiencies of 70%. Unfotunately, it has still to enter the demonstration stage. By the time Gaelectric is ready to build its plant, adiabatic CAES could be possible. However, failing that, it plans to use the best technology available now. This would give 58% efficiency and the capital cost would be $750-800/MW according to figures published by the Electrical Power Research Institute.
The best use of CAES is with floating wind turbines in deep water at sea. This is exactly what is envisaged by Professor Seamus Garvey of Nottingham University. The turbine blades would compress air directly, without electricity being produced first, and the pressurised air would pass down a pipe to what can best be described as huge polythene bags anchored to the sea floor. The heat produced by the compression would be stored and used to warm the expanding air which would turn a generator when power was needed and the electricity would be taken ashore along a high voltage DC cable.
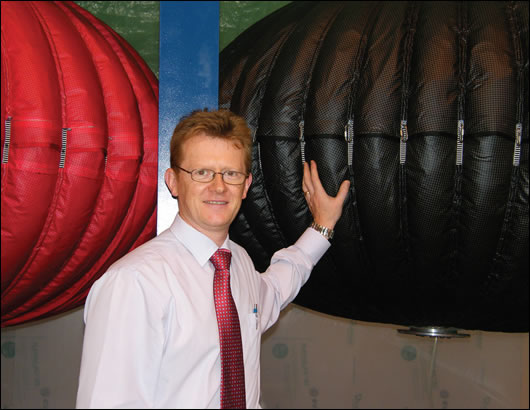
Professor Seamus Garvey with an energy bag which could store the compressed air generated by a wind turbine’s blades
Garvey's tank testing is being backed by E.on, the world's largest investor-owned energy service provider. He believes it is possible to store energy at costs well below €12,000/MWh — less than a sixth of the Spirit of Ireland figure. Storing enough energy to deliver 2GW for four days, a figure which Garvey considers a good 2020 target for Ireland, would require around 7 million cubic metres of air storage. “The optimal dimensions for energy bags are around 20m diameter and each has a volume (when full) of about 4000 cubic metres,“ he says. “For 7 million cubic metres, we would need 1750 of these bags. The seabed area covered by these would be less than one square km and the total surface area of bag material would be 2.2 million square metres.
Gravel batteries. These rely on a reversible heat pump and two insulated silos containing gravel. They store energy by using electricity to compress argon, raising its temperature to 500C. The hot gas is then passed into one silo where it heats up the gravel, thus becoming cooler itself. The gas is then allowed to expand, which cools it to around -160C, and it then goes into the other silo to cool the gravel there before going back into the compressor, effectively pumping heat from the cold silo to the hot. The electricity is recovered by reversing the process so that the heat pump, acting in thermodynamic reverse as an engine, drives an electrical generator rather than being turned by a motor.
The technology is being developed by a former UK Civil Aviation Authority engineer, Jonathan Howes. His Cambridge-based company, Isentropic, has already built and tested two prototypes u and a third is under construction at present. “The timescale for the first fully-integrated storage system is 18 months for a scaled device of 100 to 200 kW and 2.5 years for a small utility scale machine,” Howes said in April.
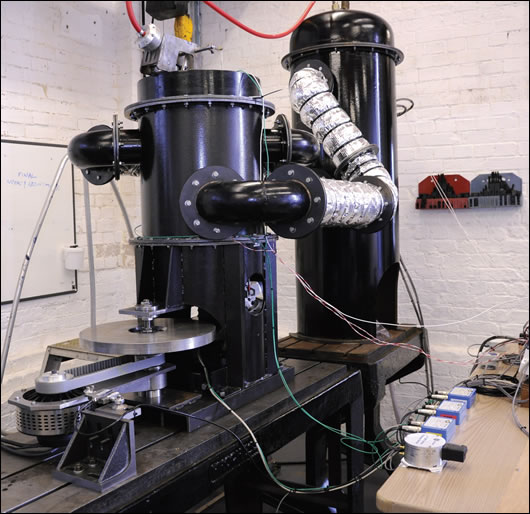
Isentropic's second prototype reversible heat engine
“The budget for development of a first utility scale plant of 2MW power with 16MWhr storage is estimated to be of the order of £10 million.” He claims a round-trip efficiency of up to 80% and that his system should be as cheap, or cheaper, than pumped storage. However its big advantage is that a gravel battery could be built anywhere and on almost any scale – it does not depend on the availability of suitable hanging valleys.
Possible solution 3: getting consumers to deal with the surplus power
Getting consumers to deal with the surplus power is the best solution of all. This is because it is much easier and cheaper for consumers to soak up the surplus by increasing their demand than it is for storage systems to take it up or for it to be exported by a capacity-limited interconnector. But consumers will only absorb the extra power if they have a significant price incentive to do so and they have some way of knowing when it gets cheap.
Supplying power at variable prices and letting consumers know its price at any time is, of course, exactly what the government is planning to make possible with its €600 million plan to replace every electricity meter in the country. The replacements are to be “smart meters” which, rather than just recording how much power is used between one meter reading and the next, record how much power is being used in each half-hourly period and relay the figure back to ESB Networks every 30 minutes. Massive information flows are therefore envisaged when the system is rolled out nationally.
Consumer tests are going on at present and in some of them, price information is being relayed to the meter using mobile phone technology but the prices are not used in the meter itself. They are displayed instead on an “in-home display unit” which 1,200 of the 6,000 customers in the trials have been given to test. The units show the amount of power they are using at the moment, its price and how much they have spent on electricity so far that day. The only smart aspects of the meter itself are that it can report voltage drops and other problems with the quality of supply back to the grid controllers and it can also turn a consumer's power on and off by remote command.
The weakest aspect of the current test programme involves getting price information to the customer in a form he or she can use. Part of the problem is that the technology for doing this does not exist yet. The display units, although among the most advanced available, do not make it easy for the customer to make informed decisions and turn appliances on and off according to sudden variations in the price of power.
The other part of the problem is that the CER believes that the public is looking for simplicity and that, as a result, the companies selling power to consumers will not charge prices based on the wholesale price which varies, as I said, at 30-minute intervals. The CER assumes that only big power users will be charged this way and that smaller consumers will be asked to choose between the various companies' “price plans” which will charge the same fixed rates for blocks of hours each day, every day.
If it is right, consumers will not get cheaper power when the wind is blowing and will be unable to benefit from it. However, if just one distributor begins charging smaller consumers prices based on the wholesale rate, all sorts of possibilities would open up. The consumers who signed up would be able, in effect, to do a lot of storage themselves by using power when it was cheap to heat water, charge the battery of their electric car or give the freezer a blast. They would also time-shift – turning the washing machine on when the price of power fell below a figure they had programmed into their in-home display unit, for example.
At the moment, the wholesale price of power varies hugely – but predictably – through each 24-hour period. For example, on October 11, 2010, the minimum price was €33.01 per MWh and the maximum was €113.73. Jerry Sweeney, who runs a data centre near Cork, has calculated that an ordinary customer who was able to buy and sell at those prices would be able to heat their house for free. They would charge up batteries at the low price, discharge at the higher one and the inefficiencies in the process would warm the area where the batteries, inverter and transformer were kept.
In-home display units (IHDs) need to be developed which would enable customers to carry out this sort of activity. They need to be smart, not the meter. As well as showing how much power the household is using at the time and the cost, advanced IHDs should display what each appliance in use at the time is costing and allow the consumer to set the time and/or the price level at which it should be turned off and on. They should also be able to link via the internet to commercial services which predict the price of power for the next few days based on time of day and expected air temperatures and wind speeds. This would enable consumers to delay electricity-hungry activities like charging their electric car until the time the wind speed on the west coast was forecast to pick up.
Studies around the world have shown that, automatic, pre-programmed responses to changes in power prices are several times greater than those which rely on letting the consumer know of the change personally and relying on him or her to take action. It is therefore crucial to have equipment that enables consumers to give a pre-programmed response. To achieve this, an electricity distributor needs to start selling power to the public at the wholesale price plus a margin. This would enable consumers who are keen to minimise their costs to buy IHDs which make pre-programming possible from manufacturers competing to supply to most user-friendly features, software and displays.
The CER would be happy for this to happen and is designing the system to make it possible. “We don't want any blockers in place,” says Paul O'Neill, who runs the smart meter programme.
But the three solutions we have looked at need to be combined. A European supergrid is necessary to achieve the “spatial redistribution” of surplus wind energy. Any of the four methods of energy storage mentioned are required to achieve its “temporal redistribution”. And, if an over-investment in interconnection or large-scale storage is to be avoided, both need to be supplemented by demand side management through variable pricing and the installation of equipment that makes it easy for customers to respond to sudden price variations. There's a fourth requirement too – the public must be paid the current wholesale price less a margin whenever it generates power itself or stores it and sells it back. The emerging electricity market needs to be a two-way street.
- Articles
- renewable energy
- Search for a store
- wind
- energy
- natural resources
- Pumped Storage
- Flow Batteries
- Compressed Air Energy Storage
- CAES
- Spirit Of Ireland
- Gravel Batteries
Related items
-
The world energy crisis 2022
-
Grant heat pumps at centre of NI energy transition project
-
SEAI Energy Awards 2020 open for entries
-
The first oil crisis
-
The Jodrell Bank grand challenge
-
SEAI Energy Show back at RDS on 27 & 28 March
-
Energywise Ireland open new renewables showroom
-
Together in Electric Dreams
-
Use all your solar electricity at home rather than export it — Warik Energy
-
SEAI Energy Show to take place on 6 & 7 April
-
Passive House Plus editor explains passive house to RTE's Eco Eye
-
Passive House Plus back issues now freely available online